Analog Discovery 2 Chart
Analog Discovery 2's high-level block diagram is presented in Fig. The core of the Analog Discovery 2 is the Xilinx® Spartan®-6 FPGA (specifically, the XC6SLX16-1L device). The WaveForms application automatically programs the Discovery’s FPGA at start-up with a configuration file designed to implement a multi-function test and measurement instrument. May 18, 2016 A Comparison of the Analog Discovery and Analog Discovery 2 May 18, 2016 May 17, 2016 - by Kaitlyn Franz - Leave a Comment There have been a lot of questions regarding the differences between the Analog Discovery 2 and the Analog Discovery.
A Zener diode is similar in construction and operation to an ordinary diode. Unlike a conventional diode where the intended use is to prevent current in the reverse direction, a zener diode is mostly used in the reverse region above the breakdown voltage. Its I vs, V characteristic curve is similar to ordinary diode. By adjusting the doping of the P and N sides of the junction, it is possible to design a Zener diode that breaks down at anywhere from a few volts to a few hundred volts. See Figure 2. In this breakdown or zener region the diode voltage will remain approximately constant over a wide range of currents.
The maximum reverse-bias potential that can be applied before entering the Zener region is called the Peak Inverse Voltage (PIV) or the Peak Reverse Voltage (PRV). A Schottky barrier diode uses a rectifying metal-semiconductor junction formed by plating, evaporating or sputtering one of a variety of metals onto n-type or p-type semiconductor material. Generally, n-type silicon and n-type GaAs are used in commercially available Schottky diodes. The properties of a forward biased Schottky barrier diode are determined by majority carrier phenomena. A conventional PN junction diode's properties are determined by minority carriers.
Schottky diodes are majority carrier devices that can be switched rapidly from forward to reverse bias without minority carrier storage effects.The normal current vs. Voltage (I/ V) curve of a Schottky barrier diode resembles that of a PN junction diode with the following exceptions:1. The reverse breakdown voltage of a Schottky barrier diode is lower and the reverse leakage current higher than those of a PN junction diode made using the same resistivity semiconductor material.2. The forward voltage at a specific forward current is also lower for a Schottky barrier diode than for a PN junction diode. For example, at 2 mA forward bias current a low barrier silicon Schottky diode will have a forward voltage of 0.3 volts while a silicon PN junction diode will have a voltage of 0.7 volts. This lower forward voltage drop can cut the power dissipated in the diode by more than one half.
This power savings can be very significant when the diodes need to carry large forward currents.The current vs. Voltage (I/ V) relationship for a Schottky barrier diode is given by the following equation known as the Richardson equation. The primary difference from the conventional diode equation is in I S with the addition of the modified Richardson constant A.Where:A = junction areaA. = modified Richardson constant (value varies by material and dopant) = 110A/(°K 2-cm 2)for n-type SiT = absolute temperature in ºKq = electronic charge = 1.6. 10 -19 Cf B = barrier height in volts k = Boltzman's constant = 1.37. 10 -23 J/ Kn = ideality factor (forward slope factor, determined by metal-semiconductor interface)LED Fundamentals.
The LED is a junction diode that emits light when forward biased. Actually all PN junction diodes emit photons when forward biased, it is just that the photons are in the infrared band and the physical shape of the diode does not allow the photons to escape the package. To achieve the visible light emitting property, it is necessary to fabricate the LED from materials with larger band-gaps other than silicon. As a result, the forward voltage drop of the LED is greater than 0.7V; usually on the order of 1.5 to 2 volts depending on the wavelength of the emitted light. The LED is also built in a special transparent package as shown in figure 3. Figure 3, Light emitting diodesAn LED is a semiconductor device that emits electromagnetic radiation at optical and infrared frequencies.
The device is a PN junction diode made from p-type and n-type semiconductors, usually GaAs, GaP or SiC. They emit light only when an external applied voltage is used to forward bias the diode above a minimum threshold value. The gain in electrical potential energy delivered by this voltage is sufficient to force electrons to flow out of the n-type material, across the junction barrier, and into the p-type region. This threshold voltage for the onset of current flow across the junction and the production of light is V 0.
The emission of light occurs after electrons enter into the p-region (and holes into the n-region). These electrons are a small minority surrounded by holes (essentially the anti-particles of the electrons) and they will quickly find a hole to recombine with. Energetically, the electron relaxes from the excited state (conduction band) to the ground state (valence band). The diodes are called light emitting because the energy given up by the electron as it relaxes is emitted as a photon. Above the threshold value, the current and light output increases exponentially with the bias voltage across the diode. The quanta of energy or photon has an energy E = hf. The relation between the photon energy and the turn-on voltage V 0, is:Where:E g is the size of the energy gap V 0 is the threshold voltagef and λ are the frequency and wavelength of the emitted photonsc is the velocity of lighte is the electronic chargeh is Planck's constantLab Experiments.
The current vs. Voltage characteristics of the PN junction diode can be measured using the Discovery Board and the following connections shown in figure 4. The green boxes indicate where to connect the Discovery board. Set up the breadboard with waveform generator output, W1, attached to one end of the resistor. The 2+ scope input is also connected here. The other end of the resistor is connected to one end of the diode being measured as shown in the diagram. The 2- scope input as well as the 1+ scope input is also connected to the second end of the resistor.
The other end of the diode is connected to ground along with the 1- scope input. The waveform generator should be configured for a 100 Hz triangle wave with 3 volt amplitude and 0 volt offset. The differential input of scope channel 2 (2+,2-) is used to measure the current in the resistor (and diode).
The single ended input of scope channel 1 (1+) is used to measure the voltage across the diode (1- input is grounded). The Scope should be setup with channel 1 at 500mV per division and channel 2 set also at 500mV per division. The current flowing through the diode, I D, is the voltage measured by channel 2 divided by the resistor value (100 Ω in this example).
Analog Discovery 2 Charter School
Use the XY display mode to plot the voltage across the diode (scope channel 1) on the X axis vs. The current in the diode (scope channel 2) on the Y axis.Procedure. As was discussed in the background section on LEDs, the I/ V relationship of different color ( i.e. Wavelength) LEDs might be used to measure Planck's constant. The datasheets for your various color LEDs should list the wavelength of the light emitted. Use those numbers in your calculations.One method to consider begins with plotting the I/ V data for each color LED on a semi-log graph. Your data should approximate a straight line, indicative of the exponential nature of the current vs.
Voltage relationship. An operational definition of the threshold voltage could be the value of the bias voltage when the current reaches 0.01 mA. Extrapolate the I/ V curves to where they cross 0.01mA current and use that as the working value of V 0.Construct a table with columns for V 0, λ, and f. For each LED, use the measured value of V 0 and the value of f to determine a value for Planck's constant and enter it as a column in the table. Find the mean value of Planck's constant and its uncertainty from your experimental values. Compare to the value given earlier.Questions.
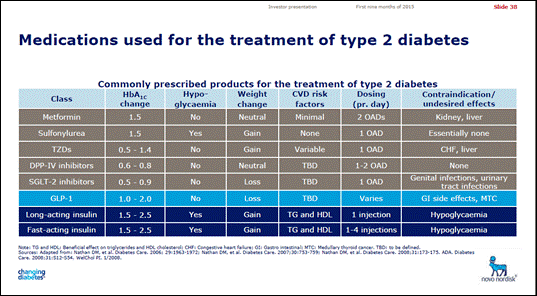
The cookies we use can be categorized as follows: Strictly Necessary Cookies: These are cookies that are required for the operation of analog.com or specific functionality offered. They either serve the sole purpose of carrying out network transmissions or are strictly necessary to provide an online service explicitly requested by you.
Analytics/Performance Cookies: These cookies allow us to carry out web analytics or other forms of audience measuring such as recognizing and counting the number of visitors and seeing how visitors move around our website. This helps us to improve the way the website works, for example, by ensuring that users are easily finding what they are looking for. Functionality Cookies: These cookies are used to recognize you when you return to our website. This enables us to personalize our content for you, greet you by name and remember your preferences (for example, your choice of language or region). Loss of the information in these cookies may make our services less functional, but would not prevent the website from working. Targeting/Profiling Cookies: These cookies record your visit to our website and/or your use of the services, the pages you have visited and the links you have followed.
We will use this information to make the website and the advertising displayed on it more relevant to your interests. We may also share this information with third parties for this purpose.